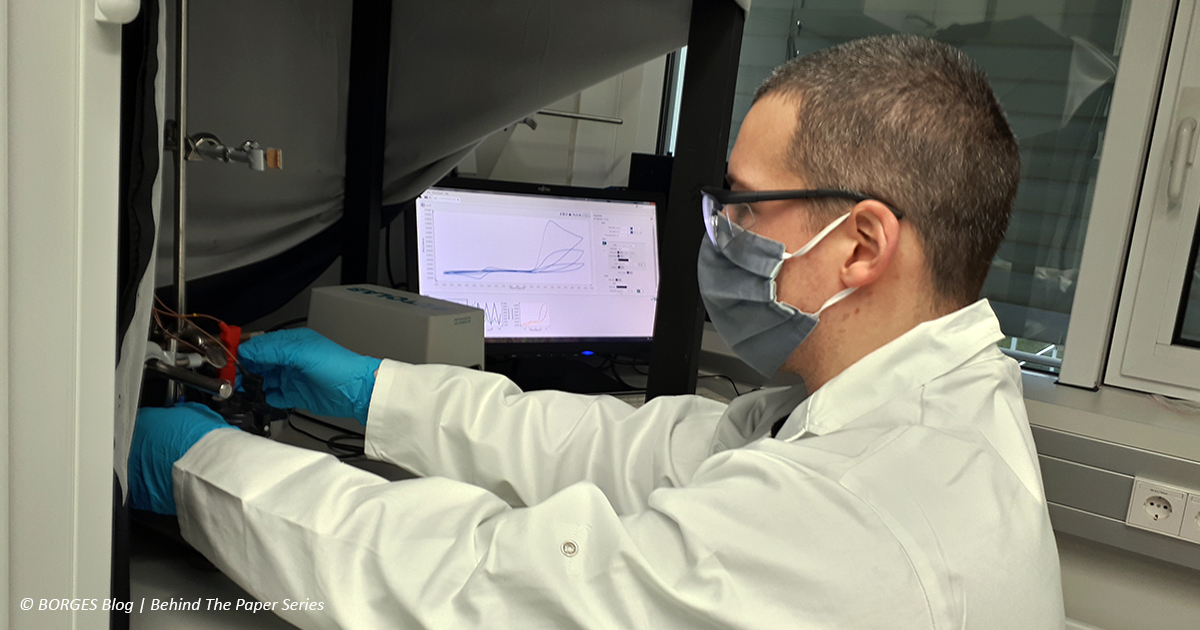
Nature has evolved cellular membranes with incredible features to control material transport to and from cells with great selectivity. Therefore, such structures are a great inspiration for nanomaterial research. The membrane proteins serve multiple roles, primarily to regulate transport processes across membranes through ligand-specificity as well as bioenergy production and the communication with the environment. There has been much interest in the fabrication of synthetic cell membrane mimics with comparable properties. Different materials have been considered including lipid bilayers, block copolymer membranes, vesicles synthetic channels or nanoporous membranes. Major efforts were made to prepare 2D structures that incorporate membrane transport proteins such as tethered lipid membranes to be used as sensors or energy production devices. However, the resulting devices were lacking in reproducibility and mechanical stability, therefore research into more stable and suitable matrix and techniques to incorporate proteins is needed.
One of the major challenges is the thickness control of the matrix. If the matrix is too thick, the embedded transport proteins will be completely covered, which limits their function as preferential transport channel. At the same time, transmembrane proteins need a phospholipid environment for maintaining their structure, and this is not offered in most of the matrices. While different types of synthetic membranes exist, they are often much thicker than natural phospholipid membranes therefore thickness control is a challenge since the transport proteins are only few nanometer thick a matrix which incorporate those would also prevents their usability by blocking the transport sites. Furthermore, due to the different chemical environment, they are not suitable to support transmembrane proteins, which require a phospholipid environment for maintaining their structure.
So-called phospholipid “nanodiscs” can stabilize transport proteins outside of phospholipid membranes, allowing to handle and study such proteins in a variety of environments. The possibility to use such nano objects to construct a cell mimic material would be of great interest to produce sensors or membranes with reduced dimensions and outstanding efficiency and maybe achieving the goal of having a material with cell membrane properties in our hands. A possible candidate would be a biocompatible matrix with a very controllable thickness and good mechanical stability to sustain the nanodiscs.
In the present study, the group of Professor Weil at the Max Planck Institute for Polymer Research developed ultrathin polydopamine (PDA) films with integrated protein pores containing phospholipids and an embedded domain of a membrane protein glycophorin A as simplified cell membrane mimics. Electropolymerization was used as a powerful technique to achieve highly controlled growth of PDA films.
Electropolymerization allows the film formation to take place on the surface of a conductive material, for example gold, by applying a potential thereby moving electrons in solution, thus oxidizing and reducing the molecules. Here, large area, ultrathin PDA films were prepared on gold surfaces with 10–18 nm thickness and dimensions of up to 2.5 cm2. Subsequently, a technique was developed to remove the intact films from the gold surface and enabling transfer of ultrathin PDA films to various other substrates such as nylon mesh, silicon or substrates containing holes in the micrometer range. The novel transfer technique gave access to freestanding PDA films that remained stable even at the air interfaces with elastic moduli of ca. 6–12 GPa, which are higher than any other PDA films reported before. As the PDA film thickness was within the range of cellular membranes, monodisperse protein nanopores, so-called “nanodiscs”, were integrated as functional entities. These nanodisc-containing PDA films could serve as semi-permeable films, in which the embedded pores could control material transport.
One of the major challenges in the work was to characterize such a very thin and heterogeneous material with a high spatial and chemical resolution. Only few techniques allows to resolve such a thin structure physically and chemically, for instance AFM-IR, a technique which combine the nanometric resolution of the atomic force microscopy (AFM) with the chemical characterization of infrared spectroscopy (IR). This technique gave the opportunity to identify the nanodiscs within the film and to map the respective position within the polydopamine matrix. In addition, the films were characterized with time-of-flight secondary ion mass spectrometry (ToF-SIMS) where the lipids mass signal was recorded from the film surface, corroborating the findings from AFM-IR measurements. These techniques confirmed that the nanodiscs were integrated into the film and accessible from the surface, i.e. they had not been overgrown with PDA during film preparation. Next, selective ion permeability of the nanodisc-containing films was analyzed. It was found that the film was highly permeable to positive ions but showed reduced permeability for negative ions likely because the nanodiscs are negatively charged. By showing that nanostructures with a protein domain can be incorporated and stabilized into a bioinspired matrix, which can also be removed from the substrate, a first preliminary step into the preparation of an artificial cell membrane mimicking material was demonstrated. In the future, these simplified cell membrane mimics may offer structural investigations of the embedded membrane proteins to achieve an improved understanding of protein-mediated transport processes in cellular membranes.

For more information, please check out our article "Ultrathin Polydopamine Films with Phospholipid Nanodiscs Containing a Glycophorin A Domain" published in Advanced Functional Materials (DOI: 10.1002/adfm.202000378).
& Stay tuned to BORGES Blog for more updates!